Specific Process Knowledge/Lithography/EBeamLithography/JEOLPatternPreparation: Difference between revisions
Line 124: | Line 124: | ||
In addition to the V30 file a .JDI file is also generated. This contains the corresponding dose modulation table. If the design is not proximity corrected the table will be empty and is not needed. If the design is proximity corrected it is necesarry to open the JDI file and copy-paste the modulation table into the JDF file used for the exposure. | In addition to the V30 file a .JDI file is also generated. This contains the corresponding dose modulation table. If the design is not proximity corrected the table will be empty and is not needed. If the design is proximity corrected it is necesarry to open the JDI file and copy-paste the modulation table into the JDF file used for the exposure. | ||
{| class = "collapsible | {| class = "collapsible" width=65% style = "border-radius: 10px; border: 1px solid #CE002D;" | ||
! width=100% | Example JDI file after PEC | ! width=100% | Example JDI file after PEC | ||
|- | |- | ||
Line 166: | Line 166: | ||
; PATTERN_SORTING_DIRECTION = XZIG | ; PATTERN_SORTING_DIRECTION = XZIG | ||
; PATTERN_WRITING_UNIT_TYPE = POSSET | ; PATTERN_WRITING_UNIT_TYPE = POSSET | ||
</pre> | </pre> | ||
Revision as of 13:29, 10 March 2023
Feedback to this page: click here
Content and illustration by Thomas Pedersen @ DTU Nanolab unless otherwise noted.
Pattern preparation for exposure on JEOL 9500
Prior to exposure a pattern must be prepared for exposure. The original pattern must be provided in GDS format. Depending on requirements and complexity level pattern preparation will involve all or subset of the following steps.
- Placement preparation
- Alignment preparation
- Bulk and sleeve separation
- Proximity Effect Correction
- Export to V30
Some steps are done using Beamer from GenISys GmbH. We advise all users to familiarise themselves with Beamer through the tutorial series found on GenISys own website. or from our own Beamer guide.
Placement preparation
Pattern placement is a bit different compared to UV mask design convention and different to how our Heidelberg MLA systems work. Pattern placement is very dependent on the boundary box of the design. All patterns are placed using the ARRAY command and they are placed with respect to the center of the design boundary box. This is illustrated with the two designs, L1 and L2, below. L1 is a design nicely centered around (0,0) with a symmetric bounding box. If placed by the ARRAY command with no offset this will come out entirely as expected. L2 is however offset from (0,0) and if exported with its local bounding box and placed by the ARRAY command with no offset, it will be exposed at the center of the substrate. Thus, it is vital to keep the bounding box in mind as the ARRAY command places patterns with respect to the center of the bounding box. To get the desired result in this example, one can define an appropriate offset in the ARRAY command for exposure of L2.
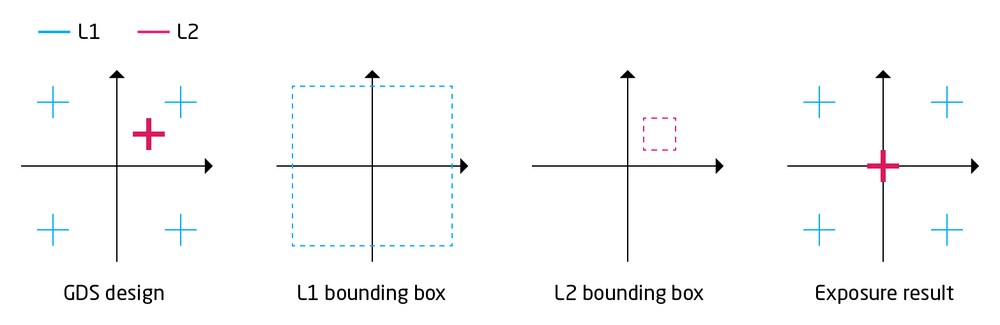
Alignment preparation
Boundary box alignment
In continuation of the example above, if two layers/exposures are to be aligned to each other in subsequent process steps it is vital that the two layers boundary boxes are identical or at least symmetric around (0,0). With reference to the example below, the boundary boxes can be made identical by either
- Letting L2 inherit the L1 boundary box (option in Beamer)
- Placing small corner marks on L2 to force the boundary box as needed
Corner marks can be 1x1 nm boxes placed outside the substrate to avoid having them exposed onto the substrate.
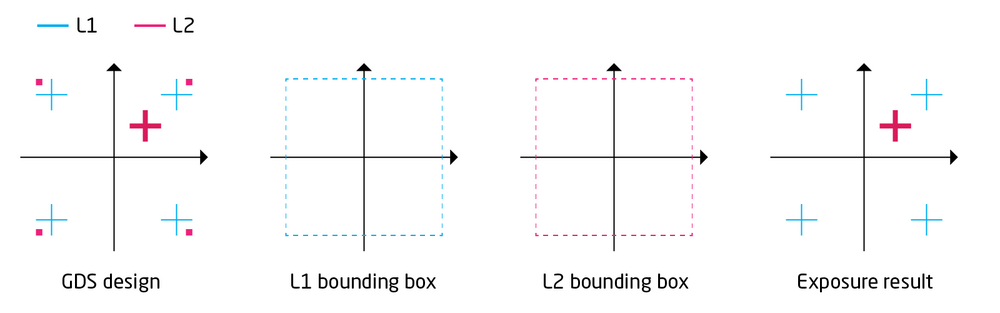
Detection of alignment mark
If alignment is needed it is vital to consider this already at the process design and mask design level to ensure that a visible mark is produced. Alignment is done using the electron beam in either SEM mode or beam scan mode. In SEM mode the stage is moved to the expected alignment mark position and the user will manually observe the SEM image and adjust stage position to center the mark at as high magnification as required by alignment tolerance of the design. In beam scan mode the user sets up a routine that will scan the beam across the expected mark position and the Backscatter Electron Detector (BED) will detect the backscattered signal. This is done in both x- and y-directions and system will calculate the mark center based on this.
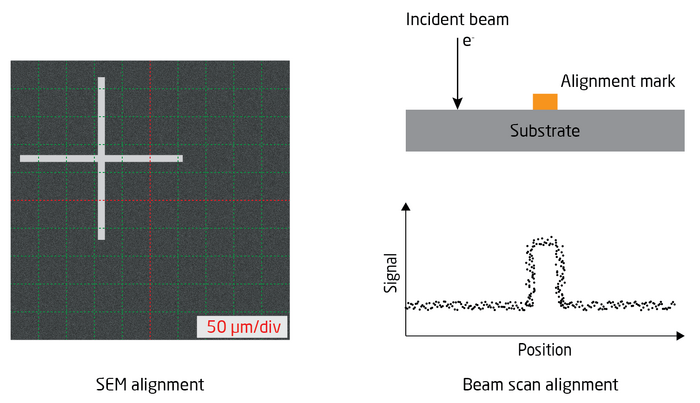
The advantage of SEM mode is that it is fairly straight forward to do. It is however also very slow as it requires constant user input to move the stage at higher and higher magnification levels until position is sufficiently determined. Due to the time it takes it is hardly realistic to do chip alignment, only global wafer alignment should be done in this way.
Automatic alignment by beam scan is significantly faster, it only takes a couple of seconds per scan. Hence this is the only realistic way of doing chip alignment. It can however be challenging to get sufficient signal on the BED for this to work if this has not already been thought into the mask and process design. Bear in mind that the electron beam is 100 kV and unless there is a relatively high difference in atomic mass between mark and substrate the signal or contrast between substrate and mark will be too low. Gold marks on Si substrates will usually work great. Deep and narrow features etched into a substrate will also work fine. Please consult with the E-beam staff or experienced colleagues when making your mark design.
Design of global marks and chip marks
As a minimum two global alignment marks must be present on the substrate for alignment. In JEOL terms these are P and Q marks. These can be used to align a full substrate design or as initial alignment of chip arrays with individual chip marks. For chip alignment either one mark (M1) or four marks (M1-M4) must be used.
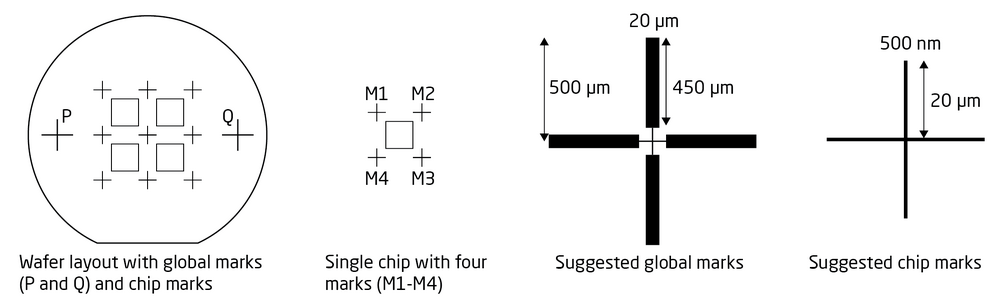
Global alignment (SETWFR) has a rough scan and a fine scan setting. Initially the mark is found using the rough scan setting, which is set to scan a long distance, typically 500 µm. Once the mark is located the machine will continue with the fine scan setting which will tyipcally scan <6 µm. In order for the machine to detect the mark on the rough scan setting the cross must have sufficient width and hence the cross is typically divided into a wide part and a slim part in the center. It is recommendable to use global marks of up to 1000 µm in height and width to make them easy and fast to find.
Chip marks are only scanned after a global alignment and hence the chip mark positions are usually very well known and the chip marks can be much smaller as indicated above.
Do NOT place any sort of text or other structures inside alignment crosses. If any other feature appear in a beam scan the system will not be able to determine which feature is the actual alignment cross.
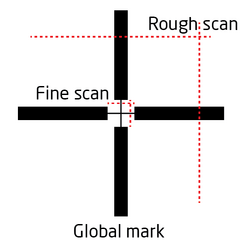
Placement of alignment marks
Global alignment marks should be placed at the left-most and right-most part of the layout. They should however not be placed closer than 15 mm to the edge of a round wafer slot as this can interfere with height sensing of the alignment mark. If alignment marks are placed too close to the edge of a wafer slot height sensing of the marks must be disabled using the HSWITCH command.
Chip marks should be placed at the corners of each chip with a gap of at least 100 µm to any important structure. Bear in mind that beam scan during alignment is a high dose exposure of the mark area and hence this resist will develop (for a positive resist). Eventhough the beam is only a few nm in diameter (dependent on exposure current) the affected area is much larger due to backscatter and proximity effect. This is illustrated in the microscope images below. On the left side is a global mark which has been scanned once in x and y rough scan and several times in fine scan mode at various locations. On the right is a chip mark that has been scanned a single time in x and y. It is obvious that the affected resist area is much larger than the scan area itself.
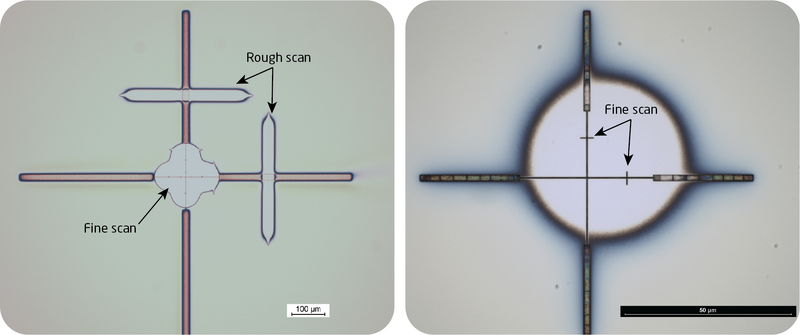
Bulk and sleeve separation - double current exposure
Patterns that contain a mix of fine and large dimension structures can advantageously be broken into a low current and a high current exposure. In this way the fine features can be written at low current (and low speed) and the large structures can be written at high current (and high speed). Some patterns, for instance a fine electrode pattern with large bonding pads, can easily and manually be broken into a fine and coarse part and placed in two different layers. For many patterns this can however be very cumbersome but the process can also be automated with Beamer using "bulk and sleeve" setup. The concept is illustrated below. In this setup, Beamer will extract the periphery (sleeve) of a pattern into one layer and the bulk of structures into another layer. The bulk is oversized slightly (adding a bias) to ensure overlap between the two patterns at exposure. In this way structures can be defined with best possible edge definition while keeping exposure time down. A Beamer flow with a default bulk and sleeve setup can be found here. A 200 nm sleeve with a 50 nm overlap is a good starting point.
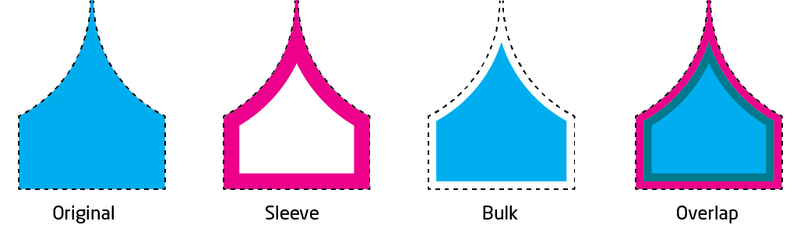
Proximity Effect Correction (PEC)
Background information
Proximity Effect Correction (or Proximity Error Correction) is a huge topic in EBL and here we will demonstrate the simplest way to apply PEC to your pattern using Beamer. For further information and more advanced uses of Beamer we advise users to go through the tutorials on GenISys own website.
The proximity effect comes from backscattered electrons that will provide a secondary exposure to the resist, as illustrated below. Thus the exposure dose received by the resist has two components; the intentional exposure from the incident beam and an undesireable exposure from backscaterred electrons. The distance of which this secondary exposure will occur is dependent on substrate material and acceleration voltage. For silicon substrates exposed at 100 kV, the distance is up to 30 µm. The amount of secondary exposure will be pattern dependent since densely populated pattern areas will suffer a lot of secondary exposure while sparsely populated areas will not suffer much secondary exposure. This will cause a local pattern bias that will depend on local pattern load. In order to circumvent this one can modulate the incident dose to account for the proximity effect. The dose modulation can be calculated with Beamer. Beamer will essentially break the design into a number of dose classes and assign each a unique dose dependent on pattern density and feature dimension.
PEC is calculated based on a Point Spread Function (PSF). The PSF is a model of the radial energy distribution when taking backscatter electrons into account. In Beamer the PSF will look similar to the plot below that shows the cummulative radial energy PSF for a silicon substrate covered byt 200 nm resist exposed at 100 kV. Notice that the x-axis is logarithmic and in this case extends to about 30 µm from the center of the beam. The red part of the curve indicates beam spread due to forward scattering through the resist.
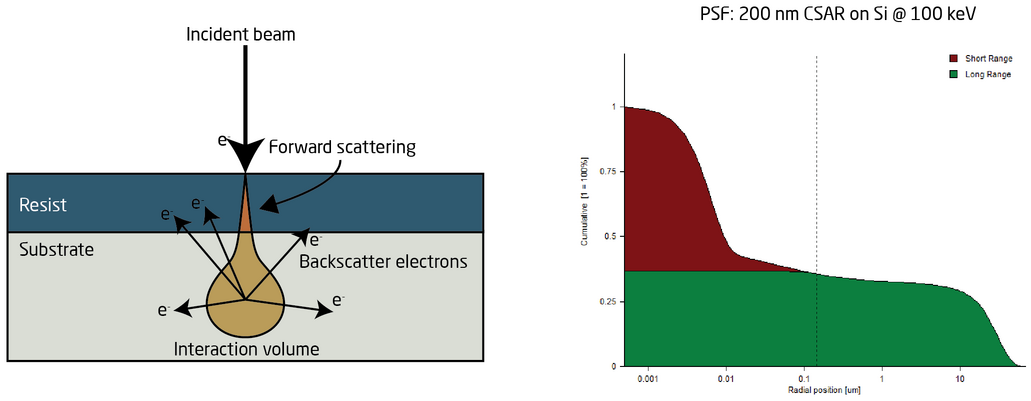
The PEC node in Beamer
Beamer provides three methods of defining a PSF:
- Load a pre-computer PSF from the archives
- Use a guassian approximation and enter the variables values
- Use a nummerical PSF
The pre-computed PSF can be loaded from either a separate file, from the local archive or from the global archive. In any case the file will probably originate from a Tracer simulation, for more on this visit our Tracer page. It can be advantageus to place your PSF in the global (network) archive such that it is available on the Beamer PC in the cleanroom as well as the Beamer PC outside the cleanroom.
A guassian approximation is mostly useful if trying to replicate a result from litterature or if one has worked deliberately towards determining the gaussian constants for a particular process.
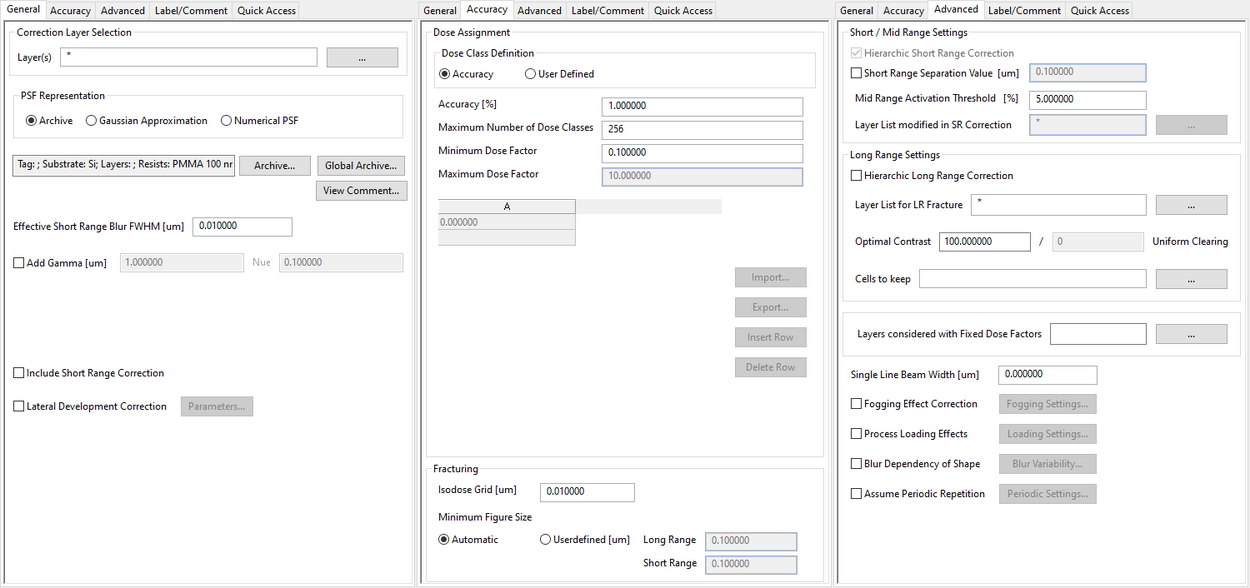
As seen above the PEC node has a lot of variables, more information can be found in the tutorials on the GenISys website, here we will only go through the most important options.
From the General tab the layer(s) to perform PEC can be selected through the Layer(s) field. The type of PSF is defined from the PSF Representation. Archived PSF's can be loaded from either the local machine Archive... or from the Global Archive... (network based and thus available on both Beamer computers). Forward scatter through the resist layer is disabled by default, it can be enabled with Include Short Range Correction at the expense of (sometimes a lot) extra computation time. Known development bias can be adjusted for in Lateral Development Correction.
In the Accuracy tab one can control the accuracy and element size of fractured elements. The Accuracy [%] field defines the maximum allowable difference in dose classes and thus has an impact on the number of dose classes generated. The Maximum Number of Dose Classes sets an upper limit to this, the JEOL 9500 system allows up to 999 dose classes in a modulation table. The Minimum/Maximum Dose Factor fields are rarely used, most designs fall into a range of 0.9-3. Under Fracturing one can control the lateral precision of fractured elements the design is broken into. Beware that if a very low Isodose Grid is set a very large number of elements can be created that will make the exposure data rate limited rather than exposure rate limited, since the computer system can only feed data to the exposure system at a certain rate. The Minimum Figure Size can be used to limit how small elements may be created, this can be used to prevent corners being broken into unnecessarily small elements.
At this point we do not have any experience with the parameters in the Advanced section of the PEC node.
Result of a PEC process
Once a PEC node has been applied to a design and executed the design is broken into a number of shot ranks. Each shot rank has a specific dose associated with it. The doses and and how they are applied to the pattern can be seen in the pattern viewer by clicking on the Color by Dose button and selecting the Dose tree. In general, large and dense strcutures will be modulated down in dose to accuont for abundant backscatter exposure while small and sparse features will be modulated to a higher dose since there is little backscatter exposure in those areas.
![]() |
Dose modulation as generated by a PEC process. |
Export to V30
As a final step to pattern preparation it must be exported to the V30 format. This is done by connecting a Export node to the flow. In the first window that opens make sure to choose V30 as the file format.
The Export node itself has quite a few options. The most import is the Machine Type field which defaults to an incorrect machine. Make sure to choose JBX-9500FS (100kV). In most cases all other options can be left at their default values. There can however be good reasons to control the fracturing and field ordering found in the Advanced tab. For information on these please check the respective sections of our Beamer guide.
In addition to the V30 file a .JDI file is also generated. This contains the corresponding dose modulation table. If the design is not proximity corrected the table will be empty and is not needed. If the design is proximity corrected it is necesarry to open the JDI file and copy-paste the modulation table into the JDF file used for the exposure.
Example JDI file after PEC |
---|
MOD001: MODULAT (( 0, -24.3 ) , ( 1, -23.6 ) , ( 2, -22.8 ) - , ( 3, -22.0 ) , ( 4, -21.2 ) , ( 5, -20.5 ) - , ( 6, -19.7 ) , ( 7, -18.9 ) , ( 8, -18.0 ) - , ( 9, -17.2 ) , ( 10, -16.4 ) , ( 11, -15.6 ) - , ( 12, -14.7 ) , ( 13, -13.9 ) , ( 14, -13.0 ) - , ( 15, -12.1 ) , ( 16, -11.3 ) , ( 17, -10.4 ) - , ( 18, -9.5 ) , ( 19, -8.6 ) , ( 20, -7.7 ) - , ( 21, -6.7 ) , ( 22, -5.8 ) , ( 23, -4.8 ) - , ( 24, -3.9 ) , ( 25, -2.9 ) , ( 26, -2.0 ) - , ( 27, -1.0 ) , ( 28, 0.0 ) , ( 29, 1.0 ) - , ( 30, 2.0 ) , ( 31, 3.0 ) , ( 32, 4.1 ) - , ( 33, 5.1 ) , ( 34, 6.2 ) , ( 35, 7.2 ) - , ( 36, 8.3 ) , ( 37, 9.4 ) , ( 38, 10.5 ) - , ( 39, 11.6 ) , ( 40, 12.7 ) , ( 41, 13.8 ) - , ( 42, 14.9 ) , ( 43, 16.1 ) , ( 44, 17.3 ) - , ( 45, 18.4 ) , ( 46, 19.6 ) , ( 47, 20.8 ) - , ( 48, 22.0 ) , ( 49, 23.2 ) , ( 50, 24.5 ) - , ( 51, 25.7 ) , ( 52, 27.0 ) , ( 53, 28.2 ) - , ( 54, 29.5 ) , ( 55, 30.8 ) , ( 56, 32.1 ) - , ( 57, 33.5 ) , ( 58, 34.8 ) , ( 59, 36.1 ) - , ( 60, 37.5 ) , ( 61, 38.9 ) , ( 62, 40.3 ) - , ( 63, 41.7 ) , ( 64, 43.1 ) , ( 65, 44.5 ) - , ( 66, 46.0 ) , ( 67, 47.4 ) , ( 68, 48.9 ) - , ( 69, 50.4 )) ; SCAN_STEP = 0.001000 ; MINIMAL_RESOLUTION = 0.000250 ; MACHINE_TYPE = JBX-9500FS (100kV) ; DIGITAL_SCALING_X = 1.000000 ; DIGITAL_SCALING_Y = 1.000000 ; MAXIMUM_BEAMSIZE = 16000 ; SHOT_SHIFT_COUNT = 1 ; OVERLAP_COUNT = 1 ; INCREMENT_SCANNER_DATA_UNIT = 1 ; SUBFIELD_SORTING_DIRECTION = YZIG ; PATTERN_SORTING_DIRECTION = XZIG ; PATTERN_WRITING_UNIT_TYPE = POSSET |